We’ve come a long way since hearing Dr. Evil of Austin Powers’ movie fame describe “a sophisticated heat beam, which we call ‘a laser’ ” to take over the world, or sitting in awe watching Jedi knights in Star Wars blast through enemies using lightsabers.
Now in real life, lasers are being used to detect cancers cells.
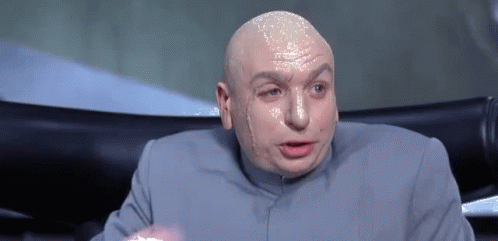
Cancer tumors have the ability to break off of their primary site and spread from their primary organ to other sites of the body via the bloodstream and lymphatic system. The spreading of cancer, known as “metastasis”, is the leading cause of cancer-related death. Although, there are currently blood tests designed to detect cancer cells in the blood, known as circulating tumor cells, these test many times cannot pick up minimal cancer cells released early on. If these current tests return as positive, this frequently means that there is a high level of cancerous cells in the blood that have spread to other organs.
However, the diagnosis and treatment of these cancer cells in the blood may soon change.
In a recent study published in Science Translation Medicine, researchers have devised a laser that can detect these malignant cells and ‘zap’ them from outside of the body. The current standard methods of detection have limited sensitivity for picking up minimal cells at early stages of the disease, therefore possibly missing an opportunity to eliminate them at a treatable juncture. A team led by biomedical engineer Vladimir Zharov, director of nanomedicine at the University of Arkansas for Medical Sciences, has developed a method in hopes of changing that modality.
In studies with melanoma, they have coupled a laser with an ultrasound detector to create a ‘Cytophone,’ a device that identifies cells acoustically.
To break it down, a laser is first shined on the surface of a person’s skin, penetrating right into some of the near-surface blood vessels. The passing melanoma cells will then ‘heat up’ because of their darker pigment and create a small ‘acoustic wave’ that then gets picked up by the ultrasound detector. Melanoma cells absorb more of the energy from the laser because of their dark pigment, allowing them to heat up quickly and expand.
This devised method can pick up a single circulating tumor cell per liter of blood, which makes this up to approximately 1,000 times more sensitive than other available methods of detection that typically examine only about 7- 8 milliliters of a sample of blood. Additionally, the cytophone was able to detect small clots of blood that could potentially grow and lead to another set of harmful consequences.
They have tested this on 28 patients with melanoma and 19 healthy volunteers.
Researchers were able to discover that within as little as 10 seconds and as long as 1 hour, the cytophone was able to detect circulating tumor cells in 27 of the 28 patients. It also did not return any false positives on the healthy volunteers. Moreover, it was found that when the energy level of the laser was turned up (still to a safe intensity) that the amount of circulating tumor cells came down over the hour, without causing any side effects.
Although the mechanism will likely not destroy all of the patient’s cancer cells, it can help in several different ways. Initially, it can be used in high-risk individuals as a screening tool to detect cancer cells in the blood. Similar to mammograms in breast cancer, it can be added to skin checks in patients that are at high risk for melanoma. While undergoing treatment, it could potentially be used to monitor the effects of that particular treatment, in addition to or separate from imaging and other blood tests, to determine if the circulating cancer cells in the blood are decreasing. Following the completion of treatment, it can be used to monitor for relapse of disease.
Even though this has been tested recently in melanoma, and the dark pigment of melanin plays a role in its detection, Zharov and his colleagues are currently working to develop methods of ‘tagging’ other cancer cells with small nanoparticles to be able to ‘heat up’ and be distinguished from the normal cells. This study holds promise but it now needs to be expanded to in a larger population including patients with a higher content of melanin. For the Silo, Jerry McGlothlin.
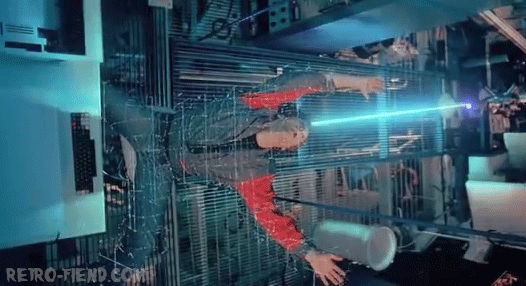
Movies aside, the future holds promise in the new hope of using lasers to fight off the evil invasions of metastasis.
About Joshua Mansour, MD…
Dr. Joshua Mansour is a board-certified hematologist/oncologist working and in the field of hematopoietic stem cell transplantation and cellular immunotherapy in Stanford, California. In June 2019 he was a recipient of the ‘40 Under 40 in Cancer’ award. Abstracts, manuscripts, and commentaries by Dr. Mansour have been published in more than 100 esteemed journals and media outlets including Canada Free Press, Today’s Practitioner, Physician’s News, and KevinMD. He has given countless presentations at conferences and other institutions, and he has helped design and implement clinical studies to evaluate current treatment plans, collaborated on grant proposals and multi-institutional retrospective studies that have been published. Joshua Mansour. M.D. has been featured on Fox Television.